Andrea Macdonald founder of ideaXme interviews Dr. Kevin Pitts, Chief Research Officer at Fermilab, America’s particle physics and accelerator laboratory and Physics Professor, University of Illinois Urbana-Champaign.
They spoke of 2 exciting research projects Muon g-2 and DUNE hosted by Fermilab which focus on muons and neutrinos respectively. Please scroll down for the interview or click the audio link above.
13.8 billion years ago matter pitted against antimatter and won. DUNE, a new experiment in the making, hosted by Fermilab attempts to discover why matter won – that is why planets, galaxies and all livings things exist at all.
The current models dictate that the Big Bang created equal parts matter and antimatter. Within a second, all the matter and antimatter should have met and annihilated, leaving behind a universe with nothing but energy in the form of light.
The first results for a second experiment from the same lab – Muon g-2 were recently published. Although, at the early stages of the experiment (5 runs in total and less than 10% of the data processed) many believe that the results to date show promise to challenge The Standard Model of Physics.
Dr. Kevin Pitts CRO Fermilab
Dr. Kevin Pitts: Kevin Pitts of the University of Illinois has been named chief research officer at Fermilab National Accelerator Laboratory beginning March 1, 2021. His focus will be on oversight for the international Deep Underground Neutrino Experiment, including advancing scientific excellence across the laboratory through strong communication, collaboration and coordination with the Department of Energy and other partners. He joins the laboratory as it prepares to usher in a new era of science and innovation in particle physics research and discovery. “We are proud to have Kevin Pitts join Fermilab at a time when DUNE is underway,” said Joe Lykken, deputy director for research at Fermilab. “His leadership and research collaboration with Fermilab make him the ideal person to direct the development of the DUNE research program both at the laboratory and with our partner institutions.” Upon its completion, DUNE, supported by the Long-Baseline Neutrino Facility, will make Fermilab the premier neutrino research institution globally. DUNE is the first international mega-science project at a DOE lab and Fermilab’s flagship project. Hosted by Fermilab, it comprises over 200 institutions in more than 30 countries. Construction work for LBNF, along with the PIP-II accelerator, are in progress at Fermilab in Illinois and at the Sanford Underground Research Facility in South Dakota. Pitts joins Fermilab after 22 years with the University of Illinois where he was most recently the vice provost for undergraduate education and a professor of physics in the Grainger College of Engineering. He has a long history working on experiments at Fermilab, including participation in the discovery of the top quark in 1995. Additionally, he has been serving on the Long Baseline Neutrino Committee since 2016. “Fermilab has been an important part of the first-half of my career and I am thrilled to be joining the laboratory as chief research officer,” Pitts said. “After numerous Fermilab research projects and collaborations, I am honored to be leading the ball down the field to further the development of DUNE and other neutrino research projects.” He received a B.A. in physics and mathematics from Anderson University and M.S. and Ph.D. degrees in physics from the University of Oregon. Pitts was a research associate at Fermilab on the CDF experiment. He also works on the Muon g-2 experiment. Kevin Pitts is a fellow of the American Physics Society, the American Association for the Advancement of Science, and is a member of the Particle Physics Project Prioritization Panel. He has received numerous awards and recognitions for his research, teaching and advising. Fermilab is supported by the Department of Energy Office of Science. The DOE Office of Science is the single largest supporter of basic research in the physical sciences in the United States and is working to address some of the most pressing challenges of our time. Official biography of Dr. Kevin Pitts, Fermilab.
Interview:
Andrea Macdonald, founder ideaXme: [00:00:00] Thirteen point eight billion years ago, matter was pitted against antimatter and matter won. We are going to be looking at the science that investigates, why. We’ll also be discussing the long awaited first results of the Muon G-2 experiment, which has received worldwide media coverage. The chief research officer at Fermilab will take us through the details of both experiments and their significance. In your words, who are you?
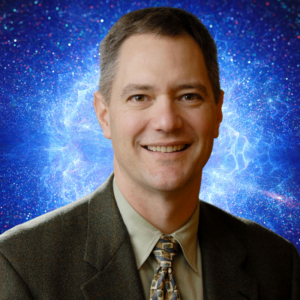
Dr. Kevin Pitts, CRO Fermilab and Physics Professor at the University of Illinois: [00:00:59] Hello, Andrea. Thank you very much for having me on your show. My name is Kevin Pitts. I’m the chief research officer at Fermilab. Fermilab is a national laboratory just outside of Chicago, one of 17 US national science laboratories. The charge for us at Fermilab is literally to understand the universe. We do this using particle accelerators and detectors that investigate particles that are coming from outer space as well. And our ultimate goal is to understand the fundamental building blocks of the universe, how everything fits together to make our world, our universe, what it is, how we got here. It actually relates very closely to philosophical questions as well as foundational scientific questions. In addition to being chief research officer, I’m also a professor of physics at the University of Illinois, which ties into one of my other passions, which is education. And so through the scientific research that we carry out, we also educate young people in areas of science and technology. One of the things I love about the work that I do is our goal is to understand nature at a really foundational level. But we develop and utilize cutting edge technology to make that happen. So, the interplay between the technology, the science and the education is truly my passion and I feel very fortunate to be able to pursue all three.
Muon g-2 Experiment Hosted By Fermilab
Andrea Macdonald, founder ideaXme: [00:02:25] Can you talk to us about the recent project out of Fermilab that has received so much coverage, the Muon g-2 experiment?
Dr. Kevin Pitts, CRO Fermilab and Physics Professor at the University of Illinois: [00:02:37] This is interesting and I think a unique result. I’ll start by just saying a little bit about what muons are. Many of your listeners and viewers will be familiar with the atom, protons, neutrons and electrons. We’ve known about this for well over 100 years and have a good explanation through the periodic table of how all these different types of atoms fit together. A lot of the questions we explore is what is inside of atoms? What are the particles that make up the particles that we’re familiar with? The electron is something we’re very familiar with. As electrons flow through wires, they light bulbs, for example, and they power computers. Well, for some reason, and we don’t know the answer, why nature decided to make copies of some of these particles that we’re familiar with. So the electron has a relative known as the muon, which has many of the same properties of the electron, but it’s actually 200 times heavier. The muon is not is not something that exists naturally as you’re walking around on the surface of the Earth, they are produced as cosmic rays from outer space interact in our atmosphere. And we can also produce muons in particle accelerators. These muons, when they’re produced, only live for about two millionths of a second before they decay away to lighter particles. They’re unstable. So, what the Muon g-2 experiment has done is to produce many, many muons. You have to produce many of them because they don’t live long and they’ve put these muons in a magnetic field.
Dr. Kevin Pitts, CRO Fermilab and Physics Professor at the University of Illinois: [00:04:11] So it sounds a little strange. I’m going to take a particle that doesn’t live for more than a couple of millions of a second. And I’m going to try to see how it behaves when I put it next to a magnet. Why in the world would I do that? And the reason is that we can predict with incredible precision how that muons should behave in the magnetic field. Forces of nature that we have not yet observed or understood yet could affect how that muon behaves very subtly. So, it’s not a drastic effect. It’s very subtle. So, the goal of the science that we’re carrying out with this experiment is to make a very, very precise prediction based on what we know. And make a very, very precise measurement based on the world as we are measuring it and to compare those two things. What we found in this case was there was a rather glaring difference between what we understand and what we know in the prediction and what we understand through the measurement. This measurement is incredibly precise. The uncertainty on the measurement. Whenever you make a scientific measurement, you have to quantify the level of precision. The uncertainty in this measurement is zero point. I won’t say all the zeros, but there are nine zeros before you get to the number five. So it’s an incredibly precise measurement. And through that precision, we can actually explore the part of the universe that has not existed naturally since a tiny fraction of a second after the Big Bang.
New Physics?
Andrea Macdonald, founder ideaXme: [00:05:46] And you talk to us about the core reasons why this has received so much media coverage and is in as far as its deviation away from the standard model or potentially?
Dr. Kevin Pitts, CRO Fermilab and Physics Professor at the University of Illinois: [00:06:01] It’s a great question, Andrea. I think there are a couple of reasons. And one of them, interestingly enough, I think is a bit historical. This is something that has been measured previously. And just like many other things that improve over time, our technology has improved. So subsequent measurements have always gotten better. This quantity was first measured back in the 1960s. The measurements we’re making now are more than ten thousand times more precise than some of the original measurements. But there was a measurement made about 20 years ago at Brookhaven National Laboratory which is in New York, and it produced what I would say was a very tantalizing hint that nature was not behaving the way we expected, but it wasn’t enough to say, voila, a eureka, we’ve discovered something new. But it raised attention. So, fast forward 20 years. A lot of hard work, a lot of improvement, both in the calculation I was telling you about, the theoretical prediction and also improvements in the experimental technique. And in some sense, the scientific world was waiting to find out. Was this interesting measurement from 20 years ago, right or not? We typically don’t say it so bluntly.
Dr. Kevin Pitts, CRO Fermilab and Physics Professor at the University of Illinois: [00:07:18] Was it right or was it wrong? But we were really waiting to see was this was this real? Was this effect real? And so this measurement now that we’ve presented recently, actually confirmed the previous measurement. So, I think that some of our collaborators who participated in that measurement 20 years ago were very relieved to find out that their original measurement was correct. And this then is further indication that nature is not behaving as we expected. Exactly what that means is yet to be seen, but it’s very exciting. And in the way this is played out historically. On top of that is the speculation associated with now you have a measurement that is very different than the prediction. And there are a number of different ways that theoretical physicists are predicting that might be explained new particles or new forces that we have not yet observed that we might be able to further understand in the coming years. So this is very exciting in and of itself, but it’s also going to give rise to another generation of experiments and calculations to further help us understand how nature works.
Andrea Macdonald, founder ideaXme: [00:08:27] There are five runs to this experiment. I believe you’re on the fourth one. Less than 10 percent of the data has been analyzed. So, there’s a lot more to do. Before we go into that and also take a look at the results from the BMW Group, can you explain for the lay people in our audience? As I explained before we went online, ideaXme’s audience is comprised of the general public as well as the leading thinkers and innovators in our world. So, for the general public, so we/they can picture it, can you talk of what this looks like? For example, the magnetic ring is 50 feet wide?
Dr. Kevin Pitts, CRO Fermilab and Physics Professor at the University of Illinois: [00:09:28] So, to produce these muons, and we need many, many muons, as you mentioned, we’ve been taking data now for almost five years. This result that I was discussing earlier was based on the first year’s data sample to make these very precise measurements of how the muon behaves in the magnetic field, especially because muons are so short lived we produce them, we accelerate them and put them into a magnetic field, but they don’t live for long. So, we have to repeat this over and over and over, literally billions and billions of times. Accumulating more data is very important to us. And we know that this result will improve with precision as we add in more data that we’ve already taken. We’re expecting to improve the precision of the measurement over the time scale of the next 12 to 15 months because that data is already available, scientists on the experiment are already analyzing that data. As you mentioned, the primary magnet that we use to measure the behavior of these muons is 50 feet or just about 15 meters in diameter. That magnet is superconducting, which means that we use electric current to produce the magnetic fields. And it’s kept very cold using liquid helium. It’s a very special magnet. It’s very unique in its nature and its construction. And in fact, one of the things that was interesting about this experiment was this magnet was used in a previous experiment at Brookhaven Laboratory in New York. And we had to move it from New York to the Chicago area to get to Fermilab. It doesn’t come apart. And so that 15 meter diameter object had to be transported intact. We had to shut down the toll way and late at night stop all traffic so that a big truck could take it on the road. It also spent some time on a barge. We had investigated at one point using a helicopter and decided that that was not the best way to go. But it was quite an undertaking just to get the experimental apparatus in place.
Andrea Macdonald, founder ideaXme: [00:11:42] Can we now talk about the big questions. You are in the first stages of this experiment. Fermilab a very open about saying that the results so far point to the potential of new physics. You are not there yet. You recognise that you are at the beginning of the process and accuracy will improve. Nonetheless, there are a number of scientists that are not so excited about this potential. Could you talk of that and the reasons why and what your counterargument to their position is?
New Physics Is Physics We Don’t Yet Understand
Dr. Kevin Pitts, CRO Fermilab and Physics Professor at the University of Illinois: [00:12:25] Yes, and I would say the first thing I would say, it’s a term we use quite commonly for your audience. I just want to clarify what we mean by new physics. New physics is physics that we as human beings don’t yet understand. It doesn’t mean that the physics has come into being recently. The universe has played by the same rules since the start. And so when we say new, it really is indicative of something that we are learning. And so, as I mentioned before, this discrepancy or this disagreement between the experimental measurement and the theoretical prediction is based on all of the science that we know is quite significant. However, these calculations, these predictions are very, very difficult to carry out. And as a consequence, many groups around the world are literally harnessing supercomputers for months on end, 24/7 to carry out these calculations. And they also use complementary approaches to carrying out these calculations. Some of them are what I would refer to as first principle calculations. What I mean by that is almost a pencil and paper type approach where you write down the things you know and you try to turn those into actual numbers. Other calculations are actually based on measurements that have been made previously of related quantities. And so there’s actually a working group put together. It’s called The Muon g-2 Theory Initiative, which is a group of theorists from around the world to try to pull together all of this information to really quantify all of the things that we know and all of the things that we don’t know.
Dr. Kevin Pitts, CRO Fermilab and Physics Professor at the University of Illinois: [00:14:01] One of the important things in science is to try to quantify what it is you don’t know. That doesn’t mean you have the answers, but you are at least trying to quantify your uncertainty. I can make a precise measurement, but how well can I make that measurement? It is a very important question. And so a number of these calculations have come together over many years to give you the prediction that I’ve been I’ve been talking about. There was a calculation that was produced by one of the research groups that has been part of this ongoing effort globally for many years recently, which produced the result that is quite a bit closer to the experimental measurement. And this is the way that science works science. We don’t just stumble upon one correct answer and move forward in lockstep. We continue to observe and then refine and learn new things and fold that into our calculations. And so, at this point, I think it’s quite intriguing because on one hand, that new theoretical prediction is quite a bit closer to the experimental measurement. At the same time, it’s rather far from some of the other theoretical predictions. And so some of my colleagues who work in the theoretical community are trying to understand this because it’s an interesting question.
Dr. Kevin Pitts, CRO Fermilab and Physics Professor at the University of Illinois: [00:15:20] Of course, first of all, it’s much closer to the measurement. And at the end of the day, the measurement is what drives us. Physics is an experimental science. You can have the most elegant mathematical theory, if it doesn’t match the data that elegant theory is not correct because the data at the end of the day is the final arbiter. So, on one hand, it’s interesting that this is closer to the measurement, absolutely interesting. But also it’s interesting that it’s rather far away from some of the other predictions. And so there’s a great deal of work going on right now to try to understand what are the differences and what are the similarities in these calculations and at the same time, kind of another generation of improved calculations at the same time. So on one hand, as I told you earlier, we’re analysing more data and this will improve the precision of the experimental measurement. On the other hand, all of this activity is going on in theoretical world. And so this is going to be an evolving story over the next year or two or three years. And so this was really an exciting in some sense, I hesitate to say, first step. As I told you, this quantity has been measured for 50 years, but it’s a step in a process. And I think the next few years of this process are going to be quite exciting.
Andrea Macdonald, founder ideaXme: [00:16:32] Through what methods? What engineering were you able to be more precise? With this experiment as opposed to the experiment 20 years ago?
Dr. Kevin Pitts, CRO Fermilab and Physics Professor at the University of Illinois: [00:16:44] One of the reasons that this measurement was able to be more precise and provided the opportunity for us to further improve precision, because as I mentioned before, we have much more data is because the particle accelerator at Fermilab is a bit more modern. And in fact, we were able to customize some of the pieces of the accelerator to maximize the number of muons that we produce. At the end of the day, the precision of the experiment is limited by the number of muons that we can measure. So, the more we can produce and put into the magnetic field and measure, the more precise this measurement will be. The Brookhaven experiment was really limited in the number of muons that it could produce. And so, through use utilizing the particle accelerator at Fermilab, we’re able to produce more particles. I should say, that Fermilab is a laboratory name is actually shorthand for the true name of the laboratory, which is Fermi National Accelerator Laboratory. Fermilab is the particle accelerator laboratory in the United States. It’s the place that the nation the United States, looks to do particle accelerator research and development. Uses of particle accelerators are actually quite interesting. Of course, we’re using them for pure science pursuits, which is one of the main reasons that a place like Fermilab exists. But accelerators exist in many aspects of society. For example, folks who receive radiation therapy for cancer treatment are utilizing very small versions of the particle accelerator that we have on site at Fermilab.
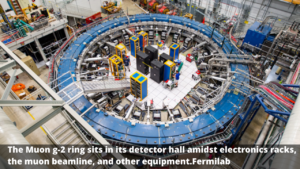
Andrea Macdonald, founder ideaXme: [00:18:29] Are you referring to the proton beam?
Dr. Kevin Pitts, CRO Fermilab and Physics Professor at the University of Illinois: [00:18:33] Proton beam cancer therapy is one example, but also x ray therapy use electron accelerators and so the techniques are very similar. The concepts are very similar, of course, that the beam, the accelerated beam or the x rays that are used for cancer therapy are much, much lower energy and aimed at killing cancerous tissue. But the concepts, the particle accelerator concepts are quite the same. The accelerators we have at Fermilab are much larger. We’re talking about kilometers in diameter to accelerate particles to very, very high energies so that we can produce these short lived states of matter that are not normally found here on the surface of the earth.
Andrea Macdonald, founder ideaXme: [00:19:23] So it’s interesting to be reminded that the general public do benefit from the millions of dollars and euros and pounds that go into these projects in as far as health and so on and so forth. It isn’t just high science, but talking of high science, can we speak about the second project, your DUNE experiment?
DUNE Experiment: How Does Matter Exist?
Dr. Kevin Pitts, CRO Fermilab and Physics Professor at the University of Illinois: [00:19:46] This is a very exciting and ambitious project that will be under development for a number of years going forward. I’ll take a step back. The DUNE project is aimed at measuring the properties of neutrinos. As I mentioned earlier, we have examples of nature making copies of itself for reasons that we have not yet been able to understand. As I mentioned earlier, the muon is a heavy copy of the electron. The electron and muon have kind of what I would call related particles called neutrinos that we are quite familiar with. The neutrinos are quite interesting because they don’t carry electric charge and they don’t interact with matter very strongly. And as a consequence, neutrinos tend to pass through just about everything without interacting at all. The sun is producing literally trillions and trillions of neutrinos every second through the nuclear reactions that the sun produces. And we see light and we see heat from the sun. We also see an enormous number of neutrinos. I shouldn’t say we see them because in fact, they pass through us. They pass through the earth on their way out into space. And so these neutrinos are very fascinating particles because they’re so elusive.
Andrea Macdonald, founder ideaXme: [00:21:12] There are three flavors of neutrinos.
Speaker2: [00:21:16] Yes, there are three flavors of neutrinos. There’s one called the electron neutrino. And that’s the one that the sun produces. And it’s very closely associated with the electron. So, again, that’s kind of the layer of matter, so to speak, that we are very familiar with. But then the muon has a related neutrino called the muon neutrino, and then there’s a third known as the tau neutrino. One of the very interesting measurements from really a couple of decades ago was experimental confirmation that there seem to be three of these neutrinos. We don’t know the reason why nature has more than one, but we also don’t know why nature seemed to stop at three. It’s very interesting. Nature replicated itself three times, but not more. These neutrinos may hold the secret, and you mentioned this at the lead into your show may hold the secret to why our universe is what I would refer to as matter dominated. Very early in the evolution of the universe, we’re quite confident that there were equal amounts of matter and antimatter. Antimatter particles are identical. Think of them as mirror particles to matter particles. So protons and neutrons and electrons we would refer to those as matter in our earth is made of and humans are made of matter. But there are things called antiprotons and antineutrons and antielectrons. It sounds quite a bit like Star Trek, and I think that kind of makes it exciting and intriguing.
Dr. Kevin Pitts, CRO Fermilab and Physics Professor at the University of Illinois: [00:22:44] There were equal numbers of matter and antimatter in the early universe. Most of the answer matter and antimatter annihilated. That is when to matter particle comes in contact with an antimatter particle they cease to exist. And what comes out is essentially light, particles of light photons, just like those that are emitted from the sun. In fact, our universe is made up primarily of light. The amount of actual particles is a small fraction of the total universe, and most of that light remains from the early universe having these matter and antimatter particles annihilate. Somewhere along the way, there became an asymmetry between the matter and antimatter. Very early on there were equal amounts, but somewhere along the line there was an asymmetry that caused a slight excess in the matter. That slight excess turned out 14 billion years later to be things like our sun, our planets, people, trees, grass, you name it. That’s the matter that was left over. So one of the very interesting and fundamental questions of modern science is: What force of nature gave rise to this slight asymmetry between matter and antimatter? We have observed examples of matter antimatter asymmetry, but we’ve not been able to identify the source that really drove the evolution of the universe. We believe that neutrinos may hold some of the answers to these questions.
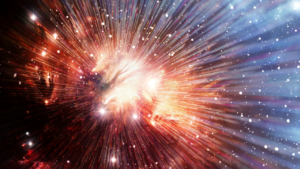
Dr. Kevin Pitts, CRO Fermilab and Physics Professor at the University of Illinois: [00:24:16] Experiments with neutrinos are extremely challenging because, as I mentioned earlier, neutrinos pass through just about everything and interact very, very rarely. You have trillions of neutrinos passing through from the sun every second. Every now and then one of them might bump into an atom in your body. It doesn’t hurt you and it doesn’t happen very often, but it can happen. And so the way we carry out experiments with neutrinos is to produce very massive detectors. They’re very large and massive so that we improve the chances that a neutrino passing through will actually bump into something. And tell us a little bit about what’s going on. The DUNE experiment takes this to another level. We’re going to produce a beam of neutrinos at Fermilab. So imagine that your source of neutrinos is in Chicago. And then what we’re going to do is send the beam through the Earth to an underground abandoned gold mine about eight hundred miles fifteen hundred kilometers away to where there will be massive detectors filled with liquid argon. Argon is normally a very heavy gas. That’s not very common. We’re going to cool the argon down to a very low temperature and have thousands of tons of liquid argon a mile underground, almost two kilometers underground. And there we will try to catch some of these neutrinos. Now, the reason for this long distance is because the neutrinos have this fascinating property that they can actually transmute from one into the other.
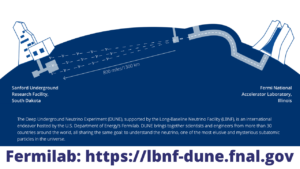
Dr. Kevin Pitts, CRO Fermilab and Physics Professor at the University of Illinois: [00:25:57] As you mentioned earlier, there are three neutrinos. We will produce a beam of muon neutrinos at Fermilab. By the time they get to the detector very far away, some of those will have transmuted into another type of neutrino. And the rate at which that happens is something that can tell us, we hope, about how the universe evolved. It’s fascinating technology. Imagine producing a beam of particles, shooting it through the earth at a target that is literally several thousand kilometers away underground and then trying to measure the properties of those neutrinos as they interact in your gigantic underground detector. The reason we put the detector in an old gold mine, so far underground, is to protect it from all of the cosmic radiation that is hitting the atmosphere. That cosmic radiation is hitting the atmosphere all the time. We have particles from outer space that are literally bumping into the earth. The reason that the cosmic radiation doesn’t hurt humans is twofold. One is our atmosphere is a nice protector. The other is that our earth’s magnetic field helps to protect us from those cosmic rays. But when you have a very sensitive experiment that’s looking for these rare interactions, a small number of these cosmic rays can cause very challenging backgrounds. So, one of the ways we reduce that is to put it under bedrock.
Andrea Macdonald, founder ideaXme: [00:27:19] Sorry to interrupt you. Similarly, as with the muon experiment, you’re also going to be looking at oscillation and comparing the oscillation of the neutrino to the oscillation of the antineutrino note. And if there is a difference, that points to something very exciting?
Dr. Kevin Pitts, CRO Fermilab and Physics Professor at the University of Illinois: [00:27:46] That’s exactly correct. And so one of the things we will do with the accelerator is we will produce a beam of neutrinos and then we actually have the ability to produce a beam of antineutrinos so we can compare how matter and antimatter behave. And the differences, we hope, will tell us a little bit about how our universe evolved.
Andrea Macdonald, founder ideaXme: [00:28:12] Can you talk about the importance of collaboration? It was incredible to discover and really, really very nice to discover in today’s world the extent to which you collaborate with scientists globally.
Fermilab’s Global Collaborations
Dr. Kevin Pitts, CRO Fermilab and Physics Professor at the University of Illinois: [00:28:29] It’s a great question and it’s something that I truly enjoy about the work that we do. By its very nature, the science that we’re carrying out is not science that you can do on your tabletop at your home university. We have to share facilities. So Fermilab is one example of a particle accelerator laboratory in the United States. The CERN research laboratory in Geneva, Switzerland is really a European hub for particle accelerator based science. And so scientists come from around the world to carry out experiments at these facilities. Muon g-2 collaboration has about 200 scientists, which is rather small on the scale some of these experiments. And what I think is fascinating about this is that the scientists from around the world are not only participating in the experiment, they’re helping to design and build and construct the experiment. So, for example, we have components of the Muon g-2 experiment that were designed and built in Italy, for example, in Pisa, and those were brought to Fermilab and integrated into the experiment. And so it’s a global team effort to carry out this science. I like to refer to it sometimes as science diplomacy, because even back in the days of the Cold War, when there were countries that essentially refused to speak to one another diplomatically, the scientists were collaborating on this type of research. And because this research is very science based, it tends to not be the kind of research you think of as classified research. And so the really the modus operandi of the scientists in this world are to be very open and very transparent. We want to share our results so our colleagues can learn from one another. As we were talking earlier and improve and develop the science as time goes forward.
Andrea Macdonald, founder ideaXme: [00:30:21] How does funding work? For example, the Muon g-2 project as a comparison to the DUNE project?
Funding Large Scientific Projects
Dr. Kevin Pitts, CRO Fermilab and Physics Professor at the University of Illinois: [00:30:31] This is primarily government funded research, so many governments are actually providing financial resources to help make an experiment like this happen. Traditionally, the host, the host region or the host country will put in a large fraction of money, really in support primarily of all of the infrastructure. So, for example, Fermilab is primarily funded, certainly funded by the US federal government. It’s actually the US Department of Energy that provides the funding and oversight for the accelerator facilities. And then that is supplemented by money from the governments of collaborating institutions. We have collaborators on the Muon g-2 experiment from the United Kingdom, as I mentioned earlier, from Italy, from other countries as well. And so those governments are providing resources to support those scientists and in some cases equipment as well to support the operation of the experiment. So it’s a very collaborative effort. CERN, as I mentioned earlier in Geneva, is a fantastic example of that, where you have a number of European nations that are providing resources in collaboration to help make this science happen. And it’s a great example of really leveraging investments, because really for any one of these countries that are participating in a collaborative like that, to make the investments necessary to carry out the science would be very difficult. But for them to bring the resources together so that the scientists can come together, that’s a very effective way to carry out the science.
Andrea Macdonald, founder ideaXme: [00:32:05] You’ve recently joined Fermilab, although have been working on their projects for some time. The Muon g-2 project is just one example. Could you talk about your work prior to joining Fermilab and your work in particular with students?
Dr. Kevin Pitts, CRO Fermilab and Physics Professor at the University of Illinois: [00:32:26] The first experiment I worked on as a graduate student myself was an experiment measuring the properties of electron positron collisions. We were talking earlier about matter and antimatter at the SLAC Accelerator Laboratory in California. We were carrying out an experiment where we were colliding electrons with their antimatter particles, which we call positrons or antielectrons. And that was it was a really exciting experiment. And I learned an incredible amount about science at that time. And then as my career move forward, I came to Fermilab originally after I’d earned my PhD for a post-doctoral research appointment and was able to work on a proton antiproton collider, which at that time was the highest energy particle accelerator in the world, has since been decommissioned because as time goes on and we build new and more powerful machines, we kind of continue to march forward in terms of technology. Throughout that time, I was so fortunate to be able to work with a number of mentors who helped me along the way, not only to learn the science, but also to learn about how to go about doing the science. We were talking earlier about what a team effort all of this is. And so to be able to interact in that science community and to really grow and develop is something that is valuable not only for a scientific endeavour, but for many things that that scientists go on to do. In the time since I became a faculty member at the University of Illinois, I’ve had the opportunity to support and help train a number of students myself.
Dr. Kevin Pitts, CRO Fermilab and Physics Professor at the University of Illinois: [00:34:06] It’s one of the most rewarding aspects of the research to bring in young scientists who are incredibly excited about the science that we are doing and help them learn about some of the technology and also the techniques. And one of the things I find fascinating about this is that many students that are trained as scientists continue on in careers in science, not surprisingly, but many of them move on into different directions. So, some of the students that I’ve worked with in the past are now in industry doing things like data science, other areas of technology, working in start-up companies. I talk to them quite frequently. We stay in touch and it’s just great to watch them grow and develop and to have families and lives of their own because we’ve become quite close. Also great to see all of the unique and interesting directions that they’re able to take this scientific training, you would think that some of the things we do are rather esoteric. And understanding how neutrinos behave may not be very relevant if you want to go out and work for a company and industry. But in fact, many of the tools and techniques that we develop, especially in collaborative science and communication, are extremely relevant.
Andrea Macdonald, founder ideaXme: [00:35:19] Could you talk a little of the spark that set you in the direction of physics?
Dr. Kevin Pitts, CRO Fermilab and Physics Professor at the University of Illinois: [00:35:26] Yes, I liked math and science growing up. I just thought it was fascinating. And when I got to college, I really didn’t have an idea of what I would like to do. Computers were kind of on the early edge of things like personal computing, things like that. When I got to college and I thought maybe I would like to get into computer science or robotics or something like that. And I took a physics course. It was a general physics course, first year physics course. And I think it’s rather interesting, Andrea. But the thing that got me excited was not all of the things we’re talking about, about muons and neutrinos and things like that. The thing that got me excited about physics was that it was so hard for me. It was incredibly challenging. I liked that. And I also like the fact that this was an area of first of all, it was a class I was taking, but it was an area where it wasn’t about memorization. It wasn’t about someone handing me all of the answers and me committing them to memory. It was about me finding the answers or calculating the answers. And I found that to be very exciting. And as time went on, I started to realize that from this field, learning about things like electricity and magnetism and gravitation and then asking some of these more foundational questions about how particles interact with one another and what are the fundamental forces that helped covered everything that we know and understand just got to be a true passion for me. And so it started with the challenge. And since then, it’s just been nothing but a really exciting endeavor that has allowed me, as I said before, to bring together my passion not only for the science, but also the technology and the education.
Andrea Macdonald, founder ideaXme: [00:37:13] Have you ever been involved at lower level teaching, what we call in this country primary school? It just seems to me that’s potentially something to be done at that level to draw more people into physics. What would you do if you were given that a group of nine/ten year olds to teach for a few hours? What would be the subject that you would focus on to try to draw them in?
Dr. Kevin Pitts, CRO Fermilab and Physics Professor at the University of Illinois: [00:37:43] I would focus on what we would refer to as hands on learning, and I would give them a challenge and I would give them some tools and let them be creative. We call this kind of active learning or project based learning. Just one example that immediately jumps to mind is what we call the spaghetti bridge project, and that is you give students some kind of dried pasta. You can choose whether it’s fettuccini or spaghetti and some other things, maybe some pieces of plastic or whatever. And you let them design a bridge and you tell them that their goal is to make the bridge as strong as possible and watch them, first of all, working groups and interact with one another, which, of course, is very important for many, many endeavors, but also to be creative at the same time while trying to solve a problem, because that’s really the foundation of what science is. Sometimes I think we get bogged down in the details of the complicated math and the calculation, but that the idea to be creative and to think broadly and to use an overused phrase outside the box to solve challenging problems, that’s what our young people need to be excited about, whether it’s physics or some other area of science or technology. But I think those are the kinds of things that young folks need to grow and develop.
Andrea Macdonald, founder ideaXme: [00:39:13] To have become chief research officer at Fermilab is an incredible thing, particularly given the projects that we’ve spoken about today. Can you talk to us a little bit about rich connectedness and the role that that potentially has played in your life, where you’ve connected with another human being who has helped you in some way to move your career forward?
Dr. Kevin Pitts, CRO Fermilab and Physics Professor at the University of Illinois: [00:39:43] Thank you for the question, because I will just say, as you’re saying, as chief research officer, I would say one of the most important aspects of my job is to connect with people, to understand their motivations and their needs and to communicate, ask questions and to listen closely to what it is they need to try to help move this entire endeavor forward. I’ve been so fortunate to have a number of connections throughout my career that have helped me move forward. But one that sticks out in my mind that I want to tell you about actually goes back to when I was in college myself. And although I had some fantastic mentors in science, one of the folks who played an enormous, outsized role in my growth and development was my baseball coach. I was fortunate enough when I was young to be to be an athlete. And I played baseball in college. And my baseball coach, he recruited me to the school. I went to Anderson University, which is a small school in in Midwestern United States, was just a fantastic human being. And he taught me so much about connectedness and life and how to move forward in some sense. And just a couple of things come to mind as I talk about this. You know, a baseball team, we play a lot of games each season.
Dr. Kevin Pitts, CRO Fermilab and Physics Professor at the University of Illinois: [00:41:09] We worked very hard training in the off season. And there are invariably, just like everything else in life there are highs and lows. And he was really the person who helped teach me about how to approach life. He used to say: Don’t get too high with the highs and really work your way through the lows because they won’t be low forever.
Dr. Kevin Pitts, CRO Fermilab and Physics Professor at the University of Illinois: [00:41:29] And so that perseverance that ethos, that hard work will pay off was something that that he brought to me. And also this whole concept of team. These experiments we’re talking about, we sometimes refer to as big science and big because of the scope of the experiments and also the number of participants. It’s a team sport. And he taught me how to approach team sports. Of course, we don’t think of science as a competitive sport per se, but a lot of the concepts about interacting with teammates and working together for the greater good, that not what one player does, not a baseball team make and not as one science scientist does a collaboration. And so the things that I learned from him in the context of sport have been just remarkably valuable to me throughout my career.
Dr. Kevin Pitts, CRO Fermilab and Physics Professor at the University of Illinois: [00:42:27] I’d like to look to the future now. We’ve spoken about Muon g-2 and also the DUNE project and of course, they’re going to be figuring very heavily in the future of the physics world, your life and Fermilab. But in addition to that, what do you think the future holds for physics? Maybe something we haven’t discussed yet today.
The Future Of Physics
Dr. Kevin Pitts, CRO Fermilab and Physics Professor at the University of Illinois: [00:42:55] It’s a fantastic question because we’ve learned so much about how the universe works over really, let’s say, the last century or so, that we know a lot now and we’re able to make precise calculations and make these measurements we’ve been talking about today. At the same time, there are many, many fundamental questions that we do not know the answer to. And as a consequence, there’s a tremendous drive on behalf of the physics community to continue forward. And we have a number of ideas about how we can continue to make measurements and understand the universe ranging from larger particle accelerators that we might build in the future to get to higher energies that might help us explore the unknown, to unique approaches to using taking advantage of some of nature’s accelerators. Nature in the form of things like black holes and quasars and pulsars has a lot to tell us by looking to the sky. So we will continue kind of a twofold approach. Where we will be refining our experimental techniques through particle accelerators, but also developing a new generation of astrophysical astronomy type experiments as well. One of the things that’s going to be a challenge going forward, though, as we continue to aspire to grow bigger and develop larger particle accelerators, are the realities, as we were talking earlier, about funding and resources.
Dr. Kevin Pitts, CRO Fermilab and Physics Professor at the University of Illinois: [00:44:36] Imagine a particle accelerator that has a circumference of maybe several hundred kilometers underground, underneath a fair swath of Central Europe, for example. That would be quite expensive to build and take a number of years. And so, again, do our governments have the interest and the will to continue to pursue this science when it quite honestly can be quite expensive to carry out some of these large scale projects? I would argue that the investment is wise not only not because the immediate consequence is it drives the economy, but the technology that we developed and the training that we’re doing is absolutely helping to move the world forward in multiple dimensions. As human beings, we want to answer these questions, but there are side benefits to this research. But it’s going to be one of the ongoing challenges as we look to the future. I like to say it this way, Andrea. We have many more ideas than there are hours in the day or dollars in the bank. And so, we’re trying to find ways to make those ideas come to fruition in ways that are financially viable and technically viable as well.
Andrea Macdonald, founder ideaXme: [00:45:50] Kevin Pitts, thank you very much for your time and thank you for moving the human story. It’s been an absolute pleasure talking to you.
Dr. Kevin Pitts, CRO Fermilab and Physics Professor at the University of Illinois: [00:46:01] Thank you, Andrea.
Credits: Andrea Macdonald founder ideaXme.
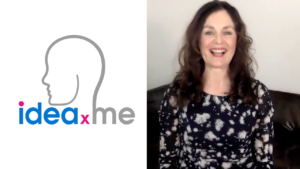
Dr . Kevin Pitts Links:
Twitter: @KevinTPitts
Linkedin: Kevin T Pitts
If you enjoyed this interview you might also enjoy our interview with Professor Winfried Hensinger, who heads the Sussex Ion Quantum Technology Group and is the director of the Sussex Centre for Quantum Technologies. He is also co-founder of Universal Quantum, a full stack quantum computing company, where he serves as Chief Scientist and Chairman. Or check out our interview with Professor Stephen Furber, the ICL Professor of Computer Engineering in the School of Computer Science at the University of Manchester.
Find ideaXme across the internet including on iTunes, SoundCloud, Radio Public, TuneIn Radio, I Heart Radio, YouTube, Vimeo, Google Podcasts, Spotify and more.
ideaXme is a global network – podcast on 12 platforms, 40 countries, mentor programme and creator series. Mission: To share knowledge of the future. Our passion: Rich Connectedness™!